Présentation
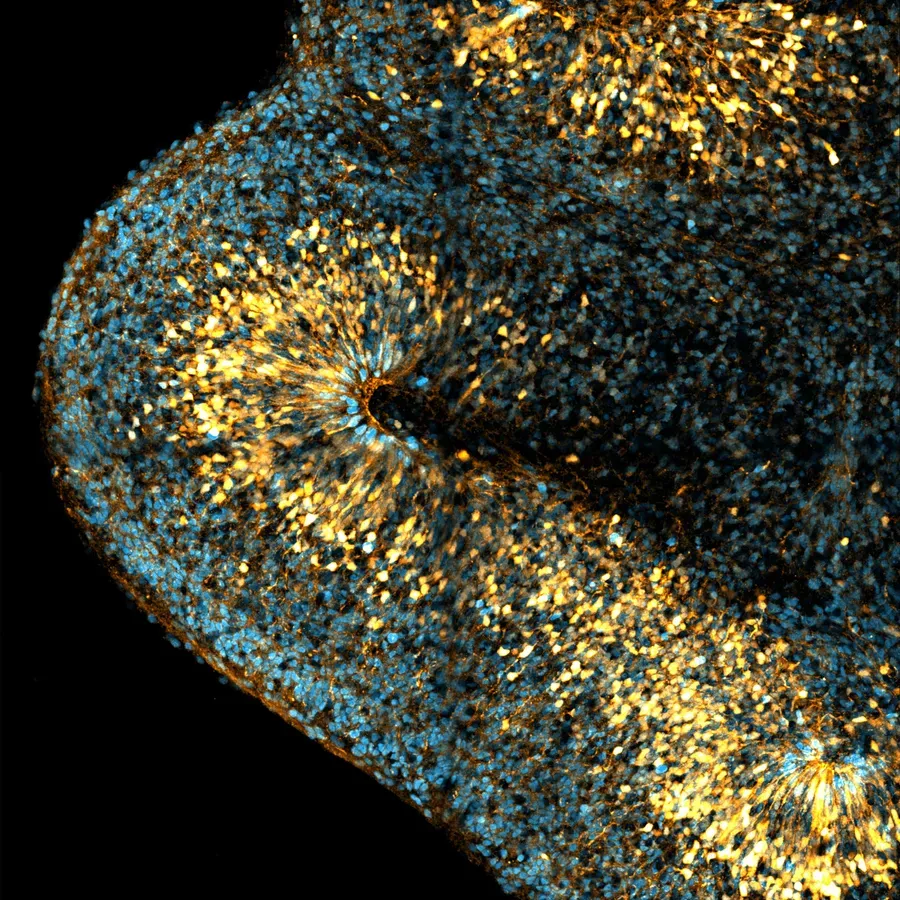
The neocortex is the center for higher cognitive functions such as language and decision-making. During its embryonic development, neural stem cells known as radial glial (RG) progenitor cells generate all neurons, astrocytes and oligodendrocytes of the neocortex. Two types of RG cells co-exist, with different relative proportions across species. Apical RG (aRG) cells are common to all mammals and localize in the ventricular zone (VZ). They are epithelial cells, anchored to the apical surface through adherens junctions. aRG cells generate neurons indirectly, through the production of an intermediate progenitor, and later on switch to gliogenesis. Basal RG (bRG) cells derive from aRG cells but have delaminated from the neuroepithelium. As such, they are non-epithelial and localize more basally, in the outer sub-ventricular zone (OSVZ). bRG cells undergo a unique form of migration, called mitotic somal translocation (MST), during which their soma very rapidly translocates just before cytokinesis. The abundance of bRG cells varies greatly across species. They are for example very rare in mouse, where they moreover may have limited proliferative properties. They are particularly abundant in certain primates, and especially in humans, where they are believed to account for their massive neocortex size expansion.
Neocortex development. aRG cells are highly elongated stem cells, present both in mouse and human. They contact the brain ventricles apically (down) and the pial surface basally (up). bRG cells are extremely abundant in human but very rare in mouse. They have lost connection to the apical surface and are localized basally. Both aRG and bRG cells can generate the different neuronal subtypes, astrocytes and oligodendrocytes. Neurogenic divisions can occur through the generation of an intermediate progenitor. |